Why do lithium-ion batteries die? (long) April 19 2016, 9 Comments
Preface
This is a very long (10,000 word, 60 image) blog post transcribing a lecture by professor Jeff Dahn from Dalhousie University titled "Why do lithium-ion batteries die, and can they be immortal?"
Not only is Jeff at the top of his field (he seriously is one of the leading figures in lithium-ion batteries), he also has a knack for making complicated things simple. The subject at hand really is technical, but anyone should be able to follow along thanks to Jeff's incredible understanding of the subject matter.
Out of the many resources we have come across on Battery Bro, this lecture stands out as one of the best.
Unfortunately, the lecture has never been transcribed, and there are no lecture notes or presentation files available anywhere on the internet. The camera-man does not always focus on the slides, and having the information in text with static images brings many benefits. Therefor we have undertaken the rather gigantuous task of transcribing the whole lecture, and here it is for you.
If you would like to contact Jeff, you can:
- email jeff.dahn@dal.ca
- call at 902-494-2991
After this lecture was made, Jeff joined Tesla Motors on an exclusive 5-year agreement which will start in June 2016. Read about it in Fortune - Meet Tesla's new weapon a battery scientist.
Watch the whole lecture if you have time
TLDR (Too long, didn't read)
If you don't have time to read or watch the whole lecture, I will do my best at condensing the meat of the article in as little words as possible for you.
- It's hard to know how long a lithium-ion battery will last.
- There are parasitic reactions that kill the batteries.
- The parasitic reactions are caused by a solid forming on the negative electrode that stops lithium-ions from moving through (plating).
- There is something called "slippage" that can be used to test for these parasitic reactions.
- Basically, when the voltage curve moves to the right after each charge-discharge cycle... this is because some lithium does not return (it becomes that solid "junk" instead).
- You need a custom-built super high-precision machine to pick this up... that Jeff and his team built.
- Manufacturers add chemicals (eg. electrolyte additives) to improve cells. Testing whether the additives are good or not takes a ton of time. But there are certain methods and machines that can significantly lower this testing time. That results in significantly faster lithium-ion battery improvement.
- Some additives are "magical" and can add a 20-fold increase to performance. So finding what stuff to add, to stop the parasitic reactions, is fundamental to improving cell life.
Beginning transcription
It's my pleasure to be here and thanks for coming.
This is a collaborative effort between a bunch of us at Dalhousie and researchers at 3M and Medtronic Energy and Component Center and E-One Moli Energy in Maple Ridge. And I would say this works been sorta going on since about 2008 roughly.
So here's a picture of the Tesla Model S Motor Trend Car in the year in 2013. It seats seven people... amazing car, really very beautiful. It's all-electric, with a 265 mile range between charges, and costs about eighty five thousand dollars. A lot of that cost is in the battery.
How long do lithium-ion batteries last?
And one of the questions people have is, even though the battery comes with an eight year warranty - it's going to be very expensive to replace, and how long will it last?
What electric vehicle manufacturers and lithium-ion battery makers know is that lithium-ion batteries struggle when the temperature is hot. So this is gonna be perhaps problematic in hot climates.
The first issue with lifetime with lithium-ion batteries in electric vehicles has come up now. In October of 2012, Nissan Leaf buyers in Southern California and Arizona sued Nissan in a class action lawsuit. They accuse Nissan of concealing Leaf vehicles that have a design defect that causes them to prematurely lose battery life and driving range. (eg. the “thermal management defect”).
So these typed things you'll see on this slide in the next few slides are directly cut and pasted off of the lawsuit that you can get online. Nissan failed to disclose and/or intentionally omitted to reveal a design defect in the leaf called the thermal management defect.
Other electric vehicles equipped with lithium-ion batteries are equipped with active thermal management systems and the idea is to keep the temperature in the battery pack down. Nissan however opted not to include an active thermal management system in the Leaf.
Lack of an adequate active cooling system cause the batteries to suffer heat-related damage causing premature battery capacity loss. Especially for those vehicles in warm climates, which are losing over 27.5 percent battery capacity within the first one or two years of operation.
So that's not good. So this a course results in the reduction a driving range, and what I want to do is to tell you why this is happening, and what we can do about it. But you gotta have some knowledge of how temperature affects lithium-ion battery lifetime and white lithium ion batteries fail anyway.
[Minute 4:00]
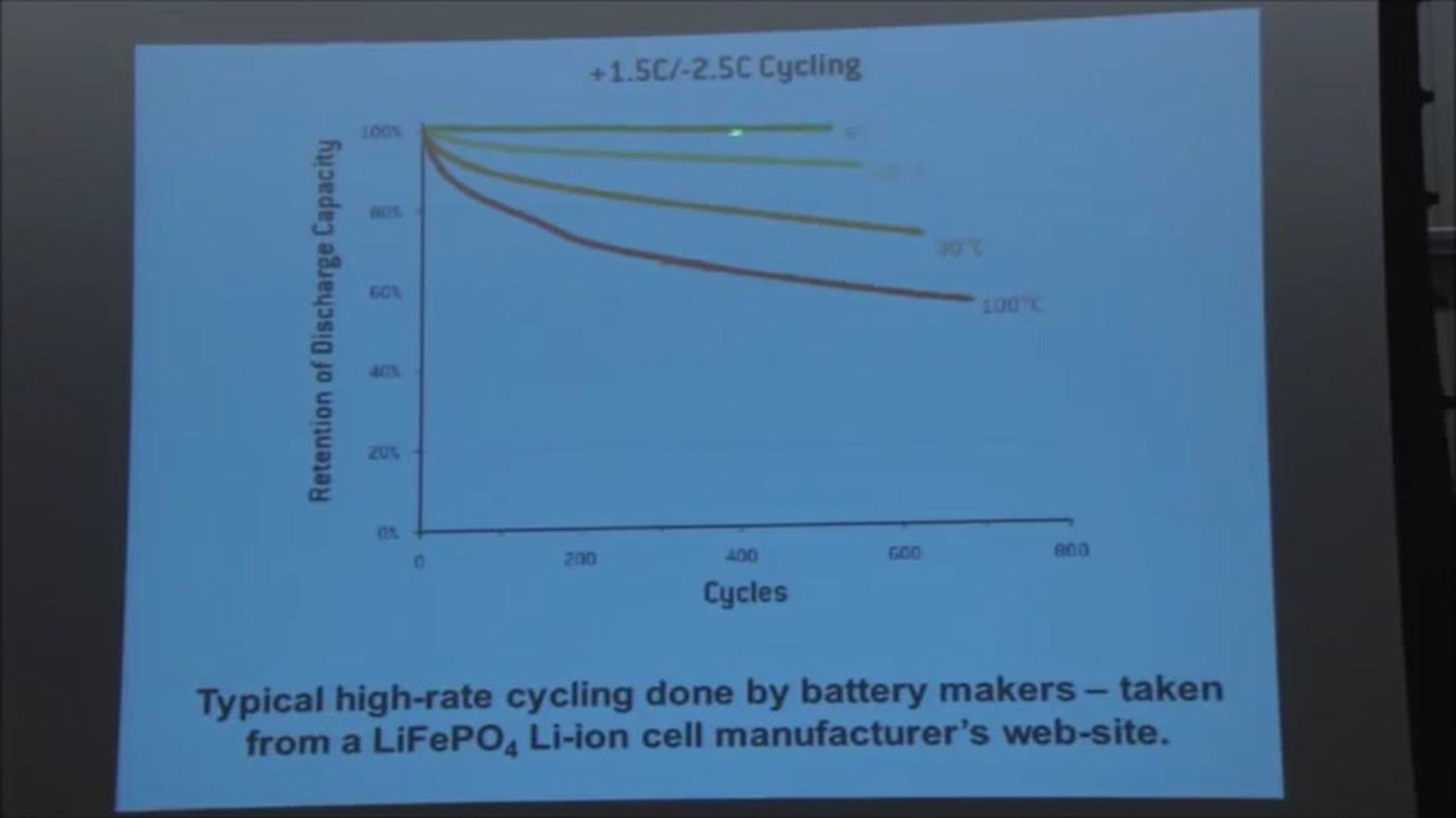
So here is a graph taken from a lithium-ion battery manufacturer’s website for lithium iron phosphate based lithium-ion cells.
It just shows the capacity that the battery delivers as a function of its charge/discharge cycle. number for cells tested at:
- Room temperature
- 60 degrees C
- 80 degrees C
- 100 degrees C (so it gets very hot)
But when you look at 60 degrees C, 500 cycles, you've only lost 10 percent of the capacity. And in Southern California and Arizona it's not sixty degrees C, and even if you're charging and discharging the battery once per day in your Nissan Leaf, you're only gonna go 300 cycles in a year.
So how the heck could you possibly be losing 27.5 percent of the capacity in the first year? Like, it doesn't make sense until you read the fine print, and the fine print is right here at the top. (+1.5C - 2.5C Cycling).
1.5 C means it was charged in about 40 minutes and this -2.5 see means it was discharged in about 20 minutes.
So each charge discharge cycle takes about an hour maybe a little more. So this entire task took about 500 hours or so.
Okay, so this experiment was done very rapidly in a little a little less than a month.
If you take the same exact cell which comes from a A123 (LiFePo4/Graphite), and you tested where you do a charge-discharge - the discharge takes 56 hours and a charge takes 56 hours (it’s the red curve). And you plot capacity versus cycle number, you can see the thing dies really fast in its cycle count.
So this blue data is the 60-degree data I showed you on the previous slide and the red data is what happens if you go much more slowly. The time a each cycle takes is much longer.
The the point is that you get a lot of capacity loss per cycle number when you go slow at high temperature because there's chemical reactions going on in the cell that are bad. And when you do charge discharge rapidly, all you're doing is beating the clock. You're beating the clock on these temperature-dependent times (time-dependent parasitic reactions.)
If you take the same data and plotted versus time, so here's the blue data for the cells cycling rapidly and here's the red for the cell cycling slowly, now they're showing more similar slopes. This really tells you that the time of exposure at high temperatures is very important to their failure.
And it shows that cells have to be cycled under realistic conditions to give a meaningful cycle and counter life determination.
Just as a point of interest, these type of batteries are not used by Tesla Motors in their vehicles. They’re used by Fisker and by GM in its Chevy Spark.
So lifetime evaluation of lithium-ion batteries is a very tough problem because if you want to demonstrate an eight year warranty, you have to test for eight years in real conditions or you have to think of something else to do.
So what the battery makers generally do is they hide in the sand and they do cycle testing.
They buy a whole bunch of chargers, and they just put cells on task for long periods of time. And it's like running sausages through a sausage factory - you don't learn very much while you're doing that.
How to accurately determine how long lithium-ion batteries will last
In 2008, we decided to really bite the bullet and invest the time and a lot of resources to maybe figure something out. We built some very high precision electronics I'll tell you about that allow us to do om measurements in a short period of time that allow us to project battery lifetime on the scale of years.
Okay so I'm gonna talk to you about experiments made an a few different types of lithium based cells. I'll talk about cells that have lithium metal negative electrode and a graphite working electrode. This is to study the behavior of the graphite electrode.
And I'll talk to you about cells that are lithium metal negative and a lithium transition metal oxide positive electrode, and that's to study the behavior the positive. And then I'll talk to you about cells that have the graphite in the positive electrode.
This is about what a lithium-ion cell consists of. These are coin cells that we make in our laboratory, and a lot of the other things I'll tell you in the lecture are on commercial lithium-ion cells made specially for us by different companies. I'll talk about the results on those too.
How exactly does a li-ion battery work?
So the first thing we have to do is understand how a lithium-ion battery works. Okay so here's a picture showing the positive electrode. A lithium transition metal oxide on an aluminum current collector. The graphite negative electrode is on a copper card collector.
These two electrodes are separated by an electrolyte that contains dissolved lithium ions. Each of these electrode materials is layered, and they’re each intercalation compounds and that means lithium atoms can reside between the layers, and they can be deintercalated and intercalated when the batteries are charging and discharging.
What's really important to recognize is the intercalation and deintercalation processes is incredibly benign that causes a structural change of about 3 percent volume change in the positive and about 10 percent in the negative. There's no structural degradation that takes place in these materials at all. The failure of the lithium-ion battery really has very little to do with structural degradation of the electrode materials during the charge discharge cycling.
When the lithium-ion battery is assembled, the negative electrode is graphite - the kind that would be in your pencil. The positive electrolyte is a lithium transition metal oxide that’s synthesized in the air at high temperature. It's stable in air. So both electrolyte materials are stable in air, you can build a battery in the open air.
[Minute 11:00]
As soon as you put it together and start to charge the battery, you force electrons in this sense to the right, the corresponding lithium-ion hops out into the electrolyte and moves to the graphite where it gets intercalated. That charges the lithium-ion cell.
Once the lithium ion cell is charged, now the lithiated graphite - graphite with lithium inside - is very reactive. It reacted like with lithium metal, and the lithium transition metal oxide with missing lithium is also very reactive. What happens is both are those real electrodes actually react with the electrolyte solution that they're in contact with.
And then you would say well then how do you make a battery that has any lifetime at all if the electrolyte reacts with the electrodes? But by luck and by chance, when the reaction occurs, the reaction products turn out to be... solid on the negative electrode. They form a passivating film that slows down and limits further reaction.
On the positive electrode a similar thing happens. So by luck these reaction don't destroy the battery. In fact they form passivating services that allow them to operate for many months.
It's the formation, or the presence of these parasitic reactions that are bad and lead to the the failure lithium-ion cells.
So when you do testing of a lithium-ion cell, this is what you normally do. Your discharge it and you charge it, and discharge it, charge it, etc. between fixed upper and lower voltage limits and you measure the capacity of the cell during charge/discharge cycling.
Measuring cell capacity
So the capacity when you're doing this experiment at constant current is very easy to calculate. It's the constant current times the time above the charge for the charge cycle, that gives you the charging capacity. The capacity of discharge is the current times the time of the discharge cycle, that gives you the discharge capacity.
[Minute 13:00]
For a perfect lithium-ion cell, the charge the cell delivers and the charge that you store in the cell during the charges should be exactly the same. So this ratio:
- CE = Qd/Qc = [Charge out]/[Charge in]
Coulombic efficiency - the ratio of charge the battery delivers to, that which you store should be exactly one.
- If CE = 1.0000000…, the Li-ion cell will last forever!
But the point is because of the parasitic reactions between the electrode materials and the electrolyte, this coulombic efficiency is not exactly one.
If you could measure the coulombic efficiency really accurately, you could use it to tell the magnitude of the parasitic reactions going on into the cell. That's going to be the point of this lecture is what can you learn if you measure the coulombic efficiency really accurately about cell lifetime.
What are the typical lithium-ion battery electrolytes?
So we have to know a little bit about the electrolyte in these batteries. They’re typically carbonate organic carbonates like:
- ethylene carbonate (EC)
- propylene carbonate (PC)
- dimethyl carbonate (DMC)
- ethyl methyl carbonate (EMC)
A typical battery electrolyte might be lithium phosphate hexa fluoride dissolved in a mixture of these solvents. The solvents are mixed because some of them have much lower boiling points and give good low temperature performance. Some of them form very good passivating layers on the negative electrode. So using a mixture tends to have benefits.
What is SEI?
As I mentioned, when the graphite becomes intercalated with lithium and it's exposed to electrolyte, it reacts with the electrolyte and a film of reaction products form on the surface and it's called the solid electrolyte interphase or SEI.
This is a picture from a paper by Emanuel Peled showing the sort of structure of the SEI. It's a heterogeneous mass comprising inorganic and organic components. But as this thing forms, it slows down the reaction between the lithium in the carbon and the electrolyte solution.
The reactions in the electrolyte solution and "slippage"
So you can take a look at this reaction between the lithium and the graphite and the electrolyte solution by studying a lithium metal versus a graphite cell.
In this lower panel now I've plotted the voltage of a lithium graphite cell as you add lithium to the graphite and then remove it again - it goes back and the voltage comes up again. But if you notice, you don't come back to zero on the capacity axis.
That's because some of the lithium that you've transferred to the graphite side has reacted and formed the solid electrolyte interphase. Some of the components in that solid electorate interphase were lithium oxide, and lithium carbonate.
They trap lithium atoms. So when you come all the way back, the graphite is empty of lithium but some on the lithium that you added is in that SEI and doesn't come back.
As you charge and discharge repeatedly, this voltage curve keep shifting to the right - always.
That comes about because some of the lithium is being trapped in that SEI film. So in this inside panel I just show you what's happening at cycles 1, 5, 10, 15 and 20, to the top of charge and the bottom of discharge.
Slippage
They keep moving to the right. The rate of motion slows down as the film gets thicker and more protective.
In the top panel here I'm plotting the discharge end-point capacity. So the point at the end of discharge as a function of the cycle number in the black dots.
You can see it increases rapidly and then starts to slow down. The top of charge has exactly the same behavior - it moves rapidly at the beginning and then starts to slow down.
As the film thickens and becomes more protective. We call this motion charge and discharge end-point capacity slippage. It’s called slippage because this is slipping to the right… so I’ll talk about slippage from time to time.
You can learn about these parasitic reactions by carefully keeping track of the end-points and how they slip.
Electrolyte Oxidation
The positive electrode shows a similar issue. So here's the positive electrode, and when that electrode gets charged the voltage goes quite high - 4.2, 4.3 volts versus lithium metal. It’s a very oxidizing condition. An electrolyte can be oxidized. I just show it schematically by electrolyte with a plus there, and the reason I show it like that is don't think anybody really knows what happens in detail.
Anyway then this oxidized electrolyte might migrate over to the negative, where it gets reduced and forms some film of reaction product. That's just one scenario that might happen.
Here we're gonna just look at a lithium metal versus a lithium transition metal oxide cell. Again that's being charged - thats removing lithium making the material more oxidizing, and add the lithium back, and it doesn't come all the way back to the axis
Every time you charge and discharge the curve keeps shifting to the right. More and more electrolyte gets oxidized and that oxidation of electrolyte causes the charge imbalance between charge and discharge.
The endpoints keep shifting to the right and unlike the negative electrode side, this shifting doesn't seem to slow down. It just keeps increasing.
So these reactions between the electrode materials and the electrolyte are bad. It's bad and temperature aggravates those reactions.
Just to remind you, these parasitic reactions that are going on in the cell - they’re bad - and by measuring the coulombic efficiency of the cell you can quantify the amount of parasitic reactions that are going on in the cell.
But you have to measure the coulombic efficiency very accurately because it's very close to one in good lithium-ion cells.
If you want lithium-ion cells that last 10,000 cycles for grid energy applications, it would need a coulombic efficiency of at least 99.99 percent. That means if you want to make statements about a lifetime of cells based on coulombic efficiency measures, you’ve got to measure at least to the fourth digit in accuracy and precision.
How to measure coulombic efficiency very accurately
But charger systems that you buy can't do this. So these two guys here took on the challenge of building a device that could measure coulombic efficiency very accurately.
Aaron Smith is now at Tesla Motors in charge of their battery lifetime group, and he graduated in 2012. Chris is still my group are working on this project, and they've done a really nice job.
This is a picture the machine that they built. It’s a sixty channel high precision charger system. Each of these brown boxes that you see is a precision current supply that supplies current with five digits of precision to a cell under test. And the cell under test are housed in these temperature control boxes so that the temperature is very stable and you can measure the charge and discharge capacities very accurately.
Everything's computer-controlled. The currents that these things output, even though they're very accurate and stable, we still pass those currents through precision resistors at the top of these racks and measure the voltage on the precision resistors to keep track of the current really well.
With this device we can measure coulombic efficiency very accurately.
I'll just show you some simple experiments that teach you about the Nissan Leaf.
These are experiments done on commercially made, what are called 18650 sized cell. 18650 batteries are 18 millimeters in diameter by sixty five millimeters long. It’s the size of your finger roughly. Batteries like that are made at a rate of about 3 billion per year, and they're used in low-end laptop computers.
Here we are cycling the cells at 60 degrees C, so very hot, and we're measuring coulombic efficiency versus cycle number. Cells are being cycled at three different rates.
- C over 24 means a charge in 24 hours and a discharge in 24 hours.
- C over 50 means 50 hours for a charge and 50 hours for discharge
- C over 100 is 100 hours
There are two cells being measured in each of these tests. I'll just draw your attention to the scale, the coulombic efficiency at the axis, the accuracy of the data, and the lack of scatter in most to the data.
Obviously if it takes two hundred hours for a cycle, you're going to get a data point every 200 hours in these bold crosses. If it takes 100 hours for a total cycle at C over 50 you get a data point every 100 hours.
You can notice that the coulombic efficiency of these cells depends on the charge/discharge rate. The reason for that is, it takes longer for a cycle, which means there is more time for the parasitic reactions to take place when you go slower.
If you instead plot the coulombic inefficiency which just one minus the CE (so just flip the data over) and then take a look carefully - you'll notice that the coulombic inefficiency scales 1 to 2 to 4 just like the cycle times do.
If you divide one minus the coulombic efficiency by the time of a cycle, all the data falls on a universal curve.
It’s telling you that the time of exposure is really the bad actor here in the failure of these cells at elevated temperature. Now these measurements can be used to rank all lithium-ion technologies.
I'll just show you some other measurements and it's a busy slide but easy to understand what you look at it.
[Minute 25:00]
There's data here for 36 cells.
In this panel (top-left) lithium cobalt oxide lithium-ion cells running at three different rates are being characterized, and it's one minus the coulombic efficiency over the time over cycle and it’s at 30 degrees C.
As we go from column to column we change to a nickel cobalt manganese lithium-ion battery [LiNiMnCo, NMC, or NCM] to iron phosphate lithium-ion battery [LiFePO 4, or LFP], and lithium manganese oxide lithium-ion battery. And you can look and say - well look at these universal curves… clearly parasitic reactions are worse in this technology. Then we’ve done the measurements at 40 degrees, 50 degrees, and 60 degrees C.
If you were going to select the lithium-ion cell for a hot climate, would you select this one? If you weren’t going to thermally manage it? Or would you select this one or that one?
How are the battery manufacturers doing
What did Nissan select? Nissan selects a blend of fifty percent of this and fifty percent of that in their cells and so does GM in the Chevy Volt. Nissan does not temperature control the battery pack. Lots a parasitic reactions going on at elevated temperature is going to lead early cell failure. GM does temperature control their battery pack and their packs a lasting longer than the Nissan under high temperature conditions.
Fisker, are another poor choice.
And Tesla has selected chemistries like lithium cobalt oxide that are very good.
These precision coulometry experiments, you look at them this way and they tell you a lot about competing technologies. Whereas, you know, a battery maker or a tester without access to this data have to test for a long time to come to these kind of conclusions about the severity of the parasitic reactions of the different technologies.
Now I want to use this equipment to start talking about how lithium-ion fails, what are we going to do about it, and how are we going to make it better.
All about electrolyte additives
I want to talk about what are called electrolyte additives. Every lithium-ion cell that you use, be it in your phone or in your laptop or tablet or whatever it is - the manufacturer has put in the electrolyte - some chemicals like a secret sauce that improves the lifetime of that cell, and they’re called additives.
The most famous is vinylene carbonate and it causes less electrolyte oxidation on the positive electrode as I'll show you in the next few slides. People add additives that reduce the impedance of cells and limit gassing in cells.
They add wetting agents because they’re trying to feed them through the manufacturing process as fast as possible. You add the electrolyte to the cell, and it has to fill into these porous electrodes. To add a wetting agent does that quicker. But how does that impact the cell lifetime and all kinds of other things?
A typical lithium-ion cell might have five additives in it for various purposes. Here I'll just show you the impact a vinylene carbonate.
Here are lithium cobalt oxide graphite lithium-ion cells. They're being cycled with the high precision charging equipment so as you charge and discharge you can see the voltage capacity curve again shifting to the right. This is caused by parasitic reactions taking place, causing electrolyte oxidation at the positive electrode. The thing is slipping to the right.
The cell measured at 40 degrees C but if you add vinylene carbonate to the electrolyte... back... just stops. So you really impact that parasitic reaction a lot with 2 percent by weight of an electrolyte additive. The same thing happens at 60 degrees C, there's a huge improvement in the rate of electrolyte oxidation at the positive electrode side.
[Minute 30:00]
Here is some other data, this is for lithium cobalt oxide cells with no vinylene carbonate in the electrolyte 1 percent and 2 percent. And then lithium cobalt oxide cells charged to a lower voltage. And cells that have a nickel manganese cobalt positive electrode.
What just want to show you here is that when you look at the data you can see that even one percent of vinylene carbonate really stops that slippage of the voltage curve to the right.
The way that we look at these data is not to look at curves like this, but instead to plot the charge endpoint capacity versus cycle number. I'll show you that on the next slide.
So here are the same cells on the previous panel, the lithium cobalt oxide cells charged to higher voltage and lower voltage. We’re plotting the charge endpoint capacity in percent versus cycle number.
So there's two cells here in the crosses. There's no vinylene carbonate added and you can see the end point slipping like crazy to higher capacities. When you add vinylene carbonate, either one or two percent it is very much reduced.
If you look at the coulombic efficiency and look at the scale here, and look how repeatable the pair cells are, (these are commercially made cells) without vinylene carbonate, the coulombic efficiency is poor. With vinylene carbonate the coulombic efficiency is much better. With the nickel manganese cobalt positive electrode, without vinylene carbonate it’s a much better situation.
Why is that? I don't know. And adding vinylene carbonate improves the situation over here. But the real take-home message here is if you measure capacity versus cycle number - I defy you to tell the difference between these different electrolytes from these measurements. They all look exactly the same.
You know, and this is what traditional battery testing is all about. You try to tell what's going on from discharge capacity versus cycle number and you're forced to cycle to end-of-life before you can tell what's going on. Whereas these high-precision measurements can distinguish between good and less good very quickly.
So this is using your head, and that's just "sausage factory" you know.
We've done this a few times where we take our short term measurements collected over a period of a couple weeks, and then we take the cells, and we've put them on a charger and run them for long periods of time.
So this is twenty hours for every cycle, 120 cycles, so 2400 hours of testing. And we increase the temperature too so that we could distinguish more quickly the differences between the cells. But you can see over here the cells that are best.
The top one over here, and then these blue symbols are next, and they’re next here, and the black is worst, and the red is next. The point is the short term measurements bear out in the long term.
If you're somebody like me, and you're trying to make lithium-ion cells better, and you don't want to test for a long period of time, you do these high precision measurements. You can tell - does the electrolyte additive help or hinder what you trying to do?
8-year long-term battery tests
Here is some data from Medtronic for cells that are implanted in the human body to run a pain relieval system. They have cells in the lab that have eight years of testing accumulated at 37 degrees C.
You can see six to eight cycles a day, twenty thousand cycles, eight years of testing at 37 degrees C. That's pretty impressive! Cells like this with nickel cobalt aluminum [LiNi0.8Co0.15Al0.05O2] are in the Tesla Motors vehicle. Tesla Motors uses technology that's at least this good. This is eight year old technology, the test started eight years ago, it has to be eight years old technology.
How do you make it better? Well do you want to test for eight years before you find out if it's better or not? So Medtronic is working with us to improve these cells, and what we do is we take their cells with different electrode additives that we and they specify, and we measure the coulombic inefficiency divided by the time of a cycle.
The data that I showed you on the previous slide is for the additive set in the blue box. We've identified additives that are much better than the one in the blue box. And Medtronic is taking the ones that at the bottom and sets them off for long-term cycling and they'll of course be better than the ones that, you know, that are already demonstrated after eight years of testing.
So the point is in a few weeks you can identify things that are way better than what is currently being used.
Now here comes the ultimate challenge for this method. That is that sometimes lithium-ion cells show this kind of failure that's incredibly insidious. These are nickel manganese cobalt [NMC] positive electrodes with graphite negatives.
Cells are cycling to an upper cut-off of 4.25 volts and they look really good. If you change the upper cut-off voltage to 4.35 volts they start out looking really good. Imagine this was in your car - you say “oh everything's great, I love it, I love it” then all of a sudden you can't even get out the driveway.
There is no way for a lithium-ion battery manufacturer to learn about when they get this rapid catastrophic failure except to cycle it until they get there. If this happens after three years or five years, you gotta you gotta go there to find it.
Catastrophic failure of lithium-ion cells
We believe that this roll over or catastrophic failure comes about because of electrolyte oxidation at the positive side.
There's no capacity fade significantly here at all.
Lithium is not getting consumed in SEI at the negative. By contrast what's happening is the electrolyte is getting oxidized, oxidation products moved to the negative, and they coat across the service of the negative and eventually shut the cell down.
Now, if you charge to a higher and higher voltage, you accelerate electrolyte oxidation. This catastrophic failure moves to lower and lower cycle numbers as you can see. The point of this is, this is a great test bed for our methods. Coulombic efficiency measurements should capture this and should be able to predict the onset of catastrophic failure.
We did an experiment with E1 Moli energy in Vancouver where they built one-hundred and sixty 18650 batteries for us. These cells had four cells of every type with different electrolyte additives in them. Cells were all the same except for electrolyte additives.
Electrolyte additives are listed up here [referring to legend].
VC is vinylene carbonate, VEC is vinyl ethylene carbonate. It really doesn't matter what these things are - the different electrolyte additives in use. The way the experiment went was we got to specify the electrode additives in 80 cells and they got to specify the electrolyte additives in the other 80 cells and they didn't even tell us what they were.
Their additives are are these numbers, whatever they might be, and our additives are these known things which I can tell you what they are but I'll tell you it doesn't matter for the purpose of this talk.
The point is - take a look at the first 50 cycles of data, look at the blown-up capacity scale, can you tell me which are these are gonna fail first? You can't! You have to cycle them to death.
We selected a design that will show this catastrophic failure, now I’ll describe to you how you design a cell to fail like that in a few minutes.
Well, I give you 100 cycles to look at. There are 100 cycles now - can you tell me which of these is going to last longest this year or which is going to fail first?
You can see one starting to drop out here now at the bottom.
So this is the problem - you're trying to improve lithium-ion batteries and you have to wait until the end of life before you know which is better... it's too slow.
[Minute 40:00]
How did the experiment go with those guys?
So this is what happened: the cells were manufactured and then they were shipped to us for cycling on our high precision charger. We also did some storage studies that I won't talk about.
They came to us and we gave them sixteen cycles on the high precision station and here I'm showing you data for five different electrolyte additive mixtures. Four of them are knowns, and 2UB means to unknowns blend B.
You can see the coulombic efficiencies on this scale vary. So FEC is worse and then VC, then VC + FEC then 2UB and then these triangles up here. Quite a variation. Then after we tested them - we don't have the resources to do all this long-term testing that takes a year or so - we sent them back to the manufacturer for their long-term testing.
The long-term testing was done at 2 amp discharge and 2 amp constant current constant voltage charge, so C-rate for the battery people. We continue the testing until the cells reached 1.6 amp hours.
On the blue boxes where the testing was done at the manufacturer. If you look here at the first 50 cycles, and you'd say well the diamonds are looking best and maybe the crosses. But when the cycling continues the crosses die first and the diamonds aren’t far after.
If you look at that high-precision cycling data - look at that - well the crosses should die first and they do and the diamond should die next and they do and the squares and circles and the triangles.
So it's really what you want, so how do you design a cell to make it do this?
If the reason the cell shows this dramatic rollover catastrophic failure is because electrolyte oxidation products migrate to the negative where they’re reduced and eventually shut down the negative electrode: what would happen if you really highly compact the graphite particles in the negative electrode?
The theory
Here is kind of a cartoon. This is a negative electrode, it's made of graphite particles. It's been highly compacted so the porosity is pretty small.
You’re cycling the cell, you’re getting electrode oxidation products to come across.
When they get over there they see a low potential service at the front of the electrode and they get reduced and form some solidified junk there.
The cell capacity is not going down very much, and you cycle some more and the layer of junk gets thicker and the pore openings start to gets closed off.
And you cycle so more and the pores ultimately become filled. Now it's very hard for the lithium ions to penetrate into the back of the electrode because it's blocked
Then lithium plating begins on the surface and the capacity dies.
That's what we believe happens, and when you take the cells apart at the end of life, you see a lot of evidence from lithium plating in certain parts of the electrode.
If you had a more porous negative electrode, what what happen then? Well you would get the same scenario but the pores don’t close.
If you make the same cells with less compacted negative electrodes they don't show the catastrophic failure - the failure looks like this (top-right graph).
But it's still bad for the electrolyte oxidation that is going on because you're losing electrolyte.
So let's go back to our scenario. Let's say that we need a certain amount of fill material to block these pores and let's say that on every charge discharge cycle, one minus the coulombic efficiency measures how much electrolyte oxidation products moved from one side to the other.
If you get this much material on every cycle, and you need a constant amount of material to block the pores, then one minus CE times the number of cycles to failure should be a constant.
- (1-CE)*(Cycles)=Constant
This constant is going to be proportional to the thickness of the film needed to block those pores.
So what you need to do is plot a graph of number of cycles to failure versus 1 over 1-CE and see what you get.
- So plot: cycles vs 1/(1-CE)
So we did this. Here's the cycles to 1.6 amp hours versus 1/(1 - Coulombic Efficiency) and there’s a pretty decent straight-line agreement here for the electrolyte additives that we know what they are.
It looks pretty good. There are some of them off the line but really there are only two sets or three sets that are significantly off the line. It's pretty amazing nobody's ever done this kind of thing ever before.
If you look at the positive electrode by SEM, I just picked one of the cells with VEC and FEC. After 420 cycles it failed before the cycling, and after the cycling the positive electrode looks exactly the same.
If you look at the negative electrode before and after you can see the buildup of this film of reaction products on the surface of the negative. So the square region has been expanded over here you, and see all this gunk on the surface of the negative and that's what is leading to the failure of the cell.
What about their additives, where they fall on the graph? I showed you the additives that we specified. Now I’ve changed the scale because the battery manufacturers are a lot smarter than university professors.
That's where there's fall So you take a look at some of these things, their coulombic efficiencies are similar, you know, to these guys, but the cycle life is way better!
This 4UA and 5UA differ only by the addition of one additive which is additive number five. This has 1234, this has 12345. Look at that - incredible.
How does it work?
From 5UA to the control with no additives there's a 20-fold increase in cycle life just with a few percent of a few magic ingredients. That's amazing - how does it work? Why are these points off our line?
Well our model assumes that any oxidation products which go over to the negative forms solid products that block the service of the negative.
Maybe when these guys go over, they don't form solid reaction products, maybe they're liquids, or maybe they’re gases - who knows. But then it wouldn't build up this film of reaction products that block the negative. So it's pretty mysterious.
Here's a graph that kind of summarizes that experiment. This is a graph showing you coulombic efficiency as a function of the number of electrolytes added to the cell. So there were four cell types that had one electrolyte additive and 11 cell types that had two and 10 that had three and three they had four and only one that had five.
But you can see in general the coulombic efficiency gets better and better as you go. And the corresponding cycles to failure generally gets better and better as you go.
So somehow these additives are acting in synergy in some way to improve things.
Now if you read the academic literature on electrolyte additives, people study one additive at a time. Are they going to learn anything relevant? I don't know because you have to put a bunch of them in to really do well. If you're not trying to figure out how this works, you're crazy, because this is the easiest way to improve lithium-ion battery technology. It's a way to really improve it like crazy.
More about the methods in this lecture
So people got interested in our methods - I was lucky to get an automotive partnership Canada grant with a bunch of credible partners. We've expanded our are high-precision charger facilities.
This system has a hundred channels that can do automotive scale lithium-ion cells and we're using it with GM and Magna to test their cells. This came operational in April 20th. It's a pretty nice system.
I want to tell you one last thing before we stop
Sometimes it's a problem working with industrial partners. You may know that if you've ever done it. Sometimes you would suggest an experiment and they say “we don't want to do that, it wastes our resources” and we say well the scientific value is important we must do it.
But our experiments on these high precision cyclers, you need very repeatable cells to be able to say “okay the difference between that cell and that cell is because of the electorate additives” and not because some graduate student made a crappy cell (or a professor made a crappy cell).
Yeah, my cells are really crappy. So we want to be free to do what we want on machine-made cells. We've established some links with Chinese battery makers who make pouch type lithium-ion cells that we buy dry without electrolyte in. Those come to us in lots of 2000.
The minimum I can buy is 2000 which is actually a pretty good number. We bought a vacuum sealer so we can seal these pouch cells at Dalhousie with whatever electrolyte we want. So this is a big step for us, and it's good, really good.
I want to tell you what we're doing with these things in one experiment. That is any these parasitic reactions in a lithium-ion cell should make heat and if you use a very sensitive microcalorimeter - you should be able to see the heat.
Using heat to test cells
So in our automotive partnership project we bought this microcalorimeter from TA Instruments. It's an amazing device - it has 12 calorimeter ports, so you can test 12 cells at once. We wired each of the ports so we can charge and discharge cells down in the calorimeter. So an isothermal calorimeter, all experiments were done at 40 degrees C, and it measures the heat that the battery is outputting or the heat that's flowing into the battery.
The machine works like a total charm, and it can hold these pouch cells that I'm talking about. The sensitivity is 10 nanowatts and the baseline stability is better than 500 nW over a month.
I'll tell you in a minute and show you the data on how much heat these pouch cells produce due to the parasitic reactions.
The heat that's given of an experiment like this - there's three sources.
When the lithium content in the electrodes changes, the entropy of the electrode materials change and there's a heat flow associated with those entropy changes - we're not interested in that.
Every battery has internal resistance or polarization between charge and discharge and there is a heat flow from that - were not interested in that either
The parasitic reactions due to the electrode electrolyte reactions - that's what we want to measure.
We have to be able to separate this from these other things that we don't want to measure. Now what sort of scale of heat flow are we looking about here?
So the cells store a watt hour of energy, they are 200 milliamp hours at four volts, so roughly a watt hour. The energy inside the complete electrolyte electrode reaction turns out is also about a watt-hour - that’s a rule of thumb for lithium-ion cells. The electrical energy is equal to the chemical energy that would take place if the electrodes reacted with the electrolyte.
If parasitic reactions occur at a rate that would consume all the electrolyte in a year, you would get a 100 microwatts. So 100 microwatts is bad. If you see 100 microwatts of parasitic reaction, that's very bad. Very, very bad.
Here's some experiments. So these are identical pouch cells, lithium cobalt oxide graphite pouch cells. One has control electrolyte and the others have different amounts a vinylene carbonate in them. They were the first charged and discharged in one open circuit. Then we discharge them, charge them, discharge, and charge them.
We did a very slow charge and discharge and I want to just concentrate on this range.
If you look very carefully up here, you'll notice on the heat scale, there's all kinds of stuff happening. Entropy changes, polarization, and so on, but the cells are identical so the entropy changes should be the same in all them.
The internal resistances are basically the same.
So the only difference between the heat output in these cells here is due to the parasitic reactions, and the heat from the blue and black is less than the red. So this is a crappy graph, right? That's what you would put on your screen just to see what happens. When you look at this you say when I really want to do is plot the heat flow versus the voltage. If you do that, what would you get?
I've added a data set for half-percent VC now as well.
At low voltage, 3.9 volts, all the four cells are about the same. Then there's a big entropy change due to an order-disorder transition in lithium cobalt oxide. But after that, between 4.1 and 4.2 volts - look - here's no vinylene carbonate, 0.05%, less heat, 2 and 4 percent, less heat again. And the difference gets greater with voltage.
So the vinylene carbonate is suppressing parasitic heat due to electrolyte oxidation. We saw that early on from our charge slippage measurements. You can see that at voltages above 4.1 volts or so, the impact of vinylene carbonate starts to get very important.
Look at the scale. So this is from that tick mark to that is 200 microwatts. So it's suppressing things by a border of 100 microwatts. That's important. So in a single experiment, you can learn about the voltage-dependence of the impact on the electrolyte additive or blend of electrolyte additives.
On just a single experiment. I don’t know how you can do this any other way but it all relies on having access to identical cells to do a measurement like this.
Pretty cool.
Conclusion and closing remarks
Alright, so, I’m done. I'll just make a few remarks:
High-precision coulometry and other methods like calorimetry will speed the development of advanced lithium-ion battery with longer lifetimes.
3M has been a partner with us for a long time but we have more partners now and everybody sees the value of these methods and the rest the world is catching on and moving into this area too.
But really the main part of the product is answering the questions:
- Why do these additives lead to benefits and how do they work?
- What is the service chemistry that takes place?
This is by far the hardest problem I've ever worked on in my life. It will take the rest of my career to maybe make some answers to this and I don't even know if we will.
We've got a couple techniques like this high precision coulometry and microcalorimetry and storage and impedance with surface science measurements. XPS and FTIR and other things that people want to do to learn how these additives work. This is where my group is going now, is to answer the why questions.
We built the characterization tools from an electrochemical point of view and now it’s time to do the hard stuff.
Thank you.
Questions and Answers
[Minute 58:00]
Question 1
Now when you were predicting the failure of your batteries, it takes a model where, the failure, the gas, [unintelligible] negative electrode, right? But you have data to show the batteries from the manufacturer, they are not far from the prediction, which is using that model. So do you think there are alternatives, maybe, beyond what you do in terms of a model?
Question 1: Response
This is about the simplest model you can ever come up with. I thought of that walking home one day.I wasn't writing fancy equations on the blackboard for years. I'm a simple minded guy and I like simple stuff. So when you do science, it’s good to work with a working model in mind and you say, well maybe the data is gonna fit this. And if it doesn't then you have to say “this is insufficient, right?” So you have to go beyond that.
Definitely you gotta go beyond that, right?
Question 1 Follow-up
So have you got any advancement… or?
Response:
Well I will show you one thing. I skipped over this in the lecture. So these guys here, imagine that this one is not making solid reaction products on the negative. 3UE, okay? So what would the impedance of that cell be like compared to these guys. Well there's no film of solids, the impedance should be lower.
So we measured the impedance of all these cells before we sent them back to the manufacturer for the long-term cycling.
I’ll show you the impedance of 3UE compared to everything else.
These are impedance spectrum (I don’t know if you’re familiar with this) but the thing that's important is the width of the semicircle here.
Look at 3UE! It’s the smallest by far of everything. If you look at the difference between 5UA which is here, and 4UA which is here. 5UA has a lot less impedance than 4UA, and then you go back to the previous thing [he is referring to the previous graph], and here's 4UA and 5UA is building less solid products on the surface, you know, and less impedance - there it is.
So if you make a 3D graph that includes the effect of impedance here… here's one minus one over C. If you go that way things should get better. Here's impedance, and if you go that way things should get better, and all the points fall on sort of a surface except that guy, and there is one outlier.
So I think the missing ingredient here is the surface film on the negative. Are you making a solid surface film or not? If you are not, that's what you want. You want that kind of an additive. You want to limit the amount of electrolyte oxidation, but you also want to make those solid products.
Long answer to a question.
Question 2: [Video skips question]
Question 2: Response
We haven't done any work, with these methods, with silicon in any great degree. But you're right - different additives are beneficial for silicon, for sure, than graphite. No I don't think you need to bring the power of these methods to silicon at this point. Silicon shows so much SEI growth on every cycle because of the volume change, that you can see the parasitic reactions with a traditional charger.
If you just have a lithium silicon cell going, and you measure coulombic efficiency - it'll be 98 percent or 96 percent or whatever it is, unless you’re cycling really fast.
You need these fancy chargers for silicon-based electrodes yet.
[Unintelligible follow-up question]
Silicon is light years away from having lifetimes like these cells i'm talking about. Silicon is light years away, okay? Maybe in ten years it'll be a thousand cycles and last a few years but it's light years from there now.
Question 2: Follow-up
If you used this to look at something like lithium titanium oxide, which has a much higher potential, you can see where the electrolyte actually forms, actually where the electrolyte oxidized product ends up being reduced - and at what potential does that plus get reduced?
Question 2: Response
Lithium titanate is amazing. It's because the voltage is high. I don’t have a lithium titanate slide here.
Sort of the best coulombic efficiency numbers we get for C over 20 cycling at 40 degrees C for graphite negatives and lithium-ion cells is about 9992. Lithium titanate with lithium-ion cells we would get 99998. Amazing. Lithium cobalt oxide lithium titanate cells with a 4.1 volts... amazing.
They’re going to last till she's dead. [Laughing] No doubt - even in you they’ll last till you’re dead. Even though you have a big advantage in years.
Question 3
Is there a way to relate the lifetimes in CHBC [audio not clear] measurements to something like a drive cycle? Can GM say that because this cell in an HBC measurement is 10,000 cycles that relates to 10 years?
Question 3: Response
This is what's gonna happen. So we're working with GM now. It's an experience for me because GM doesn't make lithium-ion cells - they buy them. So they haven't thought about what all this stuff means too much and they want to do what you’re saying. Say “the high percent charge says this, and all of a sudden the drive cycle says that.” So what I tell them is “this what you gotta know, what is a lifetime of your current technology and the test you currently run?”
Then we do the measurements on your current technology and whatever's coming next. And we compare, and we can tell you what's coming next is gonna be worse or better and make a projection about how much better.
But for me to go from high-precision cycling data without any other knowledge to predicting what a drive cycle is gonna do... impossible. So GM is sort of coming around now and coming to see.
You can also do things like see what's the impact of the lifetime of the drive cycle - you look at how the coulombic efficiency varies with temperature, and then you can get arrhenius laws and whatever and go back to the drive cycles that way.
So I don't really know how to answer your question well today... probably in a year or two these kind of things will have developed somewhat.
Question 4
Just a quick question. For the purposes of lifecycle testing and determining the buildup on the electrode in these things, are all cycles create equal? So it seems that when they do this battery testing, they use a constant charge rate and a constant discharge rate. But if you’re looking at an EV for example, you drive to work, it holds at a constant charge, then you drive it a little more, it’s at a grocery store so you can [unintelligible] a little more.
So your charging is not a linear constant discharge rate, right? And it is discharging sporadically throughout the day. I don’t know if this affects anything though. But it was sort of related to his last question - they’re doing constant charging and might have overnight charging. But if they’re testing it at constant charge and discharge rates, this might not be indicative of drive cycles at all. I don’t know if this affects anything.
Question 4: Response
The biggest issue is the time spent at highest voltage. The longer you spend at the highest voltage the worse it is. You could see from the calorimeter experiment things got worse when you went up above 4.1 volts. So if you go to 4.2, it’s worse than 4.1. If you go to 4.1 it's worse than 4.0. So the GM Volt for example, it charges to 80 percent which is 4.03 for that cell, which is decent. Not too much parasitic reactions going on there. But if it charged to 100 percent, it would be worse. So all cycles are not created equal to answer your question.
The more time you spend at higher voltage, the worse.
I have cells from 1999 that were stored at about 20 percent state of charge so maybe 3.5 volts for those cells. We put them on in 2013… and they are like new. Because the positive electrode side is not doing anything bad at low voltage.
But if they have been stored at high voltage it wouldn't have been nearly as good.
Question 4: Follow-up
This might be a consumer education thing - don't keep your EV topped off at all times.
Question 4: Follow-up Response
Well the the battery manufacturer's way around that is going to be to select technology that is OK at the voltage it's going to. So it's it's gonna be under control. People will sort of understand this.
Question 5: [unintelligible]
Question 5: Response
There are no redox holes in in any of the cells. There are no intentionally added redox shuttles in any of the cells, let’s put it that way. Maybe some molecule gets created in some funny way that lowers the coulombic efficiency a little bit... some shuttle action.
Question 6: [no audio available]
Question 6: Response
No I would say interest is not waning at all. All the automakers have various things underway.
Mercedes has the EVs and Diesels and all that stuff going on at the same time. BMW has a big EV project, and I think the diesel guys are excited, the gas guys are excited, and the EV guys are excited. Everybody's excited, and I'm excited!
Question 7
Can we turn to page 22? [Rest of question audio not intelligible]
First it goes slightly to the left, and then it starts going to the right. It goes left first and then to the right.
It's a pretty tiny leftward shift and a pretty tiny rightward shift.
Do you know about overhang? Overhang in lithium-ion cells? The negative electrode is slightly wider than the positive. That's because if the positive electrode were opposite nothing, and you recharge the cell, the lithium would come out and electroplate on edge of the negative and form lithium metal dendrites. So the negative electrode is wider.
That means there's extra graphite out on the overhang so some of the lithium from the positive goes over there and you often get weird things happening at the very beginning of cycling when this overhang equilibrates with the amount of lithium in it. It’s very technical, but when you look at the fine scale like you're looking - things get technical.
Question 8: [no audio available]
Question 8: Response
A combination of both, absolutely. There's a lot of folks that are in the battery companies that just test stuff. What we've been starting to do, which is just a slightly higher level is, we’re trying to see which additives are working, and say there's a core on that like a sulfur double bonded to oxygen, or a sulfur double bonded to two oxygens. And then the rest of the molecule you can change in a systematic way and see what happens.
There are people there doing theory. We need more people doing theory.
Question 9: [unintelligible]
Question 9: Response
Keep it as cool as possible at all times. Put it in the fridge at night, then it won't bother you while you're sleeping too. [laughter]
Added advantage. No but I'm serious - if you keep any battery as cold as possible it will last longer. So any lithium ion cell, you should keep in the fridge when you're not using it, it will last longer.
If you don't charge to a hundred percent that will help. But you know temperature is a bad actor - keep in the fridge. If you don't mind condensation on the keyboard when taken out.
Question 10: [unintelligible]
Question 10: Response
That stuff is not what I would call SEI. This is the the reduced oxidation products coming from the other side - it's junk. That stuff you can see it with an SEM. The normal SEI, what you would call SEI, is you know, just a few nanometers thick. You can’t see it on an SEM at this scale. This junk that has come from the positive and been reduced on the negative surface.
Comments
hulikkal on September 07 2018 at 08:25AM
Informative blog
Thanks for sharing
vihan on September 07 2018 at 08:25AM
This is really good and useful article for me. I am a regular reader of your articles. And here this one is the best one. You have covered everything . Thanks
Bill on June 28 2018 at 12:32PM
Best yet explanation! To get my 20 year lifp-battery bank life, I know now that I need a cooler for my 10 Battle Born 100ah (as of now) lifp-batteries: on a thermostat with a high of 80F and enclosure heat strips activated by a low of 59F. That sounds more manageable than waiting on academia and industry getting their $hit together (for any time in the foreseeable future!) Thanks again!
;-o
Advance Auto Parts on January 24 2018 at 08:13AM
A car battery is one of the most important parts of a car. In the sense, it is as important as the car engine, because without it, it is impossible to start the vehicle without having to push it from behind. It is the heart of the car and movement begins when car batteries function the way they’re supposed to.
Elmar on February 17 2017 at 07:45AM
Aren’t there any techniques to revive a completely dicharged lithium cell? I have done some pretty basic experiments to dicharge lithium cells completely and then trying to bring them back from complete failure. Ofcourse I don’t know what is going on, on a chemical level. Is the extra resistance when trying to charge them back coming from SEI? Why are they loosing there ability to hold voltage? I really want to figure it out.
I have tried cooking, freezing sub 20 for extended times, microwaving for short periods, putting mechanical pressure to compact the cells.
Do you have more suggestions that I could test? I really want to make some progression on this field.
Thanks in advance
tina h on December 03 2016 at 05:37PM
Thank you for transcribing this. I am looking at buying an EV in the next 4 years and wondered about age vs. use in battery life. It seems like, in this lecture at least, that it is the use of the battery — charging and discharging — that mainly contributes to failure. I’m wondering what impact age has.
For example, if you have: 1) an EV that gets driven once a week for 5 years and; 2) an EV that gets driven every day for 5 years — would the EV with significantly fewer trips/charges be in significantly better condition at the end of these 5 years?
Luke Wells on October 27 2016 at 07:55AM
Authur,
The negative electrode is probably Lithium Titanate (LTO). You can hear professor Dahn discuss this material as a negative electrode briefly in this presentation. Lithium intercalates into LTO at around 1.55v above LiM; as opposed to 50mv for graphite. So the use of LTO means that your energy density is reduced. However, since this electrode is at this higher potential, the electrolyte isn’t reduced on it. No real SEI is formed, from what I understand. Without an SEI, ions are able to move in and out of this material with less resistance. This allows you to charge faster safely.
Arthur Zakari on June 16 2016 at 11:21PM
I just purchased a super fast portable charger from www.techgetgo.com called PowerGo SS 5000. They told me they used a “special type of lithium battery”. It recharges extremely quick I was able to get a full recharge in 19 minutes give or take 2 minutes. Just wondering if anyone knows what type of battery this is.
Kody on April 19 2016 at 06:44PM
Wow, thanks for taking the time to transcribe this!